Research Topics
:
Our research topics include the following various subjects from the
Big-Bang cosmology to the particle and nuclear physics, and active
national and international collaborations are carried on among
interdisciplinary sciences of astronomy, astrophysics, cosmology,
particle and nuclear physics, meteoritic science, plasma physics, and
many others both theoretically, observationally and experimentally. |
|
|
Big-Bang
Cosmology
|
There
occurred many physical
processes in the early Universe which affect cosmic evolution. They are
the inflation and subsequent creation of elementary particles, the
cosmic phase transitions including quark-hadron transition, the
symmetry breaking of four kinds of fundamental forces associated with
baryo- and lepto-genesis, the weak decoupling of light neutrino
families, and so on. We study these fundamental processes and their
effects on the primordial nucleosynthesis, cosmic microwave background
anisotropies, and structure formation of various scales theoretically.
We aim to construct a universal view of cosmic evolution based on
fundamental physics of particles and nuclei so that our theoretical
models are established through cosmological observations and physics
experiments.
|
Particle
Cosmology, Extra-Dimension and Space-Time Structure
|
Recent
observations of cosmic
microwave background anisotropies and Type Ia supernova
magnitude-redshift relation have suggested a hypothesis that a flat
cosmology of accelerating universal expansion best explains the
observed data. What is the nature of dark energy, which manifests a
mysterious property of negative pressure and positive energy density?
What is the unseen cold dark matter, which is still undiscovered
experimentally? We try to establish the physical origin of the dark
energy and also identify the dark matter as quantum massive particles
required for the spontaneous symmetry breaking or anomaly restoration
theoretically. Brane world cosmology with extra dimensions is motivated
by a unified M-theory or superstring theory which manifests Einstein
Universe as a hyper-surface (i.e. brane) in higher dimensional
manifold. We aim to understand the space-time structure and explore the
quantum properties of cold dark matter particles such as the lightest
super-symmetric particles by studying observational signature in cosmic
gravitational wave background, cosmic microwave background radiation,
Type Ia supernova magnitude-redshift relation, baryon-to-mass ratio of
rich clusters, cluster mass-to-light ratio, etc. |
|
|
Cosmological
Magnetic Field, CMB and Structure Formation
|
Magnetic
field, in addition
to gravity, plays a critical role in the formation processes of various
stellar objects and their dynamical evolution. We study the effects of
cosmic magnetic field on the large scale structure. We calculate
theoretically the cosmic microwave background anisotropies and their
polarization by taking account of the primordial magnetic field in
order to constrain the strength and power spectrum at the photon last
scattering surface. We try to model the creation mechanism of the
primordial magnetic field in several cosmological phase transitions and
other dynamical processes.
|
Cosmic
Chemical and Dynamical Evolution
|
Evolution of
physical
conditions of the early Universe, clusters, galaxies, and stars are
represented by the growth or decline in chemical composition of metals
which originate from the Big-Bang nucleosynthesis and the later
evolutionary cyclic chain of star formation nucleosynthesis supernova
explosion matter ejection into interstellar space. We study the various
nucleosynthesis processes of the atomic nuclides such as the rapid- and
slow-neutron capture processes, alpha-process, proton-process,
neutrino-process in stellar evolution and supernovae. We apply to
describe the metal-enrichment in the galaxies and the Universe in order
to construct the best model of the cosmic chemical and dynamical
evolution. We also try to construct the best nucleo-cosmochronometer
using long lived radioactive nuclei.
|
Supernovae
and Gamma-Ray Bursts, Nucleosynthesis, and Neutrino Oscillation
|
Where is the
origin of the
rapid-neutron capture elements such as Thorium (half life 14.05 Gy) and
Uranium (4.5 Gy), which are the useful cosmochronometers, and the
super-heavy elements? We have not yet identified astrophysical sites
for the r-process uniquely although there are several proposed
candidates such as supernovae, neutron-star mergers, or collapsars
(i.e. central engines of gamma-ray bursts). We study the
nucleosynthetic origin of these heavy elements by modeling the
explosion dynamics of core-collapse Type II supernovae and gamma-ray
bursts. Both neutral and charge current interactions of neutrinos with
nuclei and induced neutrino effects due to vacuum and matter
oscillations and quantum self-interactions play the critical roles in
the explosive nucleosynthesis in these explosions. We try to determine
the unknown neutrino-oscillation parameters in terms of nucleosynthesis
of light-to-heavy elements in the neutrino-processes. We also study the
mechanism of pulsar kick of proto-neutron stars and gravitation wave
emission. We propose astronomical observations and particle-nuclear
physics experiments in order to test our theoretical models.
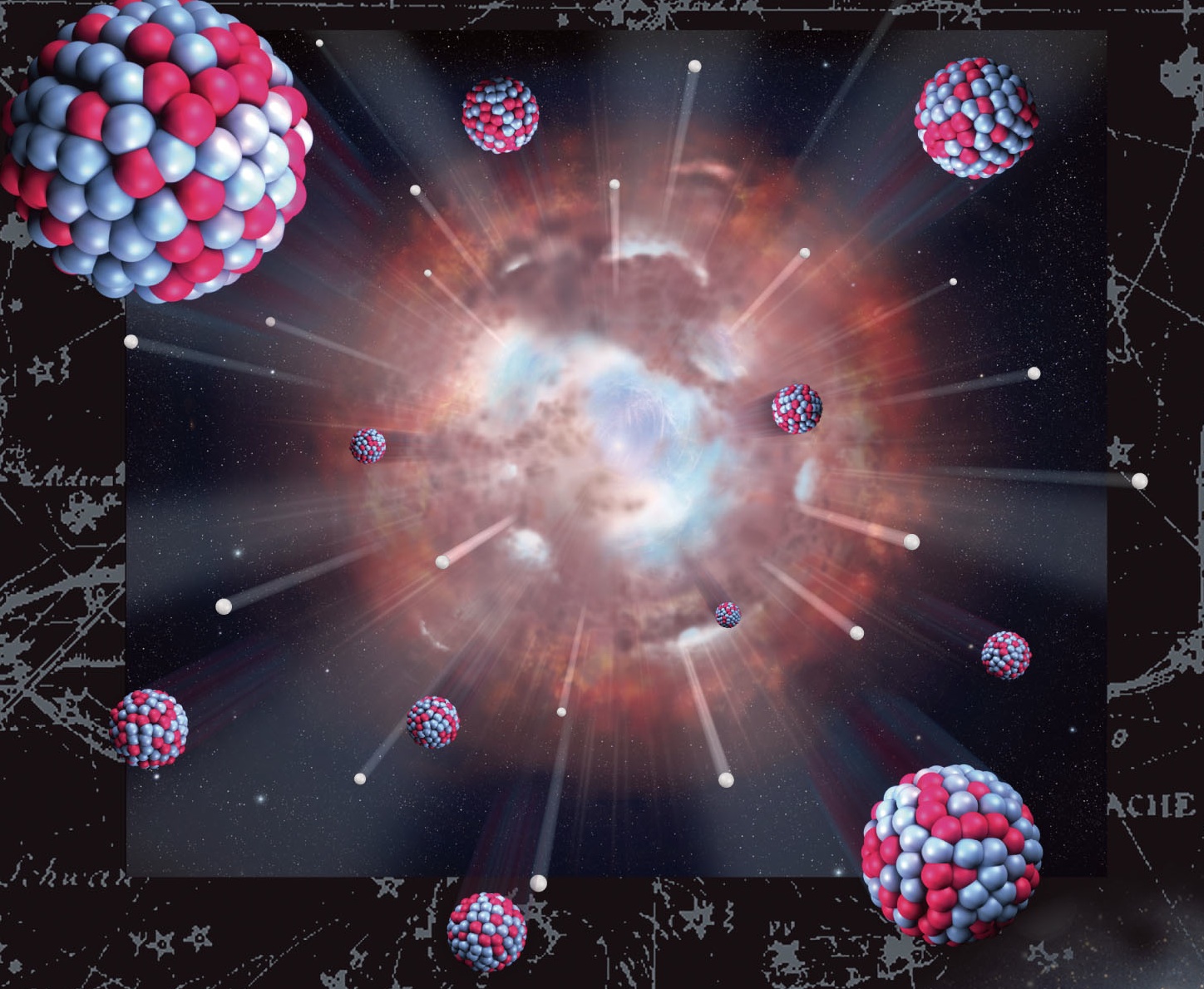
|
Galactic
Cosmic Rays and Rare Elements
|
Rare
light-to-heavy mass
elements have several different origins; the Big-Bang nucleosynthesis,
the Galactic cosmic-ray interactions with interstellar medium, the
supernova gamma- and neutrino-processes, and the AGN jet interactions
with gas clouds. We study the production mechanism of these elements in
high-energy Galactic cosmic-rays or AGN jets by modeling realistic
source spectra and their propagation through the cold gas clouds. We
aim to limit these origins quantitatively in order to constrain the
most complicated and fundamental physical processes of supernova gamma-
and neutrino-nucleosynthesis. |
Ultra-High-Energy
Cosmic Rays and Active Stellar Objects
|
What
is the origin of
ultra-high-energy cosmic rays? What is the realistic picture of the
central engines of gamma-ray bursts? Strongly magnetized compact
stellar objects such as rapidly rotating proto-neutron stars or black
holes are the viable candidates. We study the interactions of
ultra-high-energy charged particles (hadrons) with strong magnetic
fields on these relativistic compact objects in quark models.
Quantizing the meson field in the constituent quark model, we calculate
the meson synchrotron radiation probabilities in strong magnetic field
and study the implication in the ultra-high-energy cosmic rays whose
energies exceed 10^20 eV. |
|
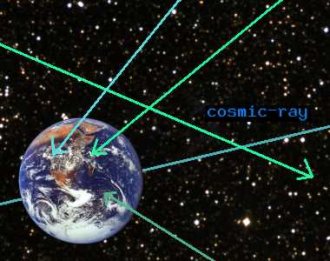 |
|
Major Research
Subjects of
the Members :
|
Michael Famiano (Professor, Western Michigan University)
|
For relativistic stellar plasmas, electrons and positrons are created via pair production. Nuclear reactions in stellar environments may then be enhanced by the presence of electron-positron pairs. Preliminary results indicate that this screening effect may enhance reaction rates above the currently utilized "classical" screening model. However, relativistic effects have never been studied. We hope to explain and enhance the commonly used classical screening model.
The primary goal is to produce a systematic comparison of the effects of relativistic electron-positron production in hot stellar environments to the currently-used classical models. These results will provide better input to understanding X-ray bursts, core-collapse supernovae, massive stellar burning, and type Ia supernovae light curves and nucleosynthesis. |
Roland Diehl (Professor, Max Planck Institut fuer extraterrestrische Physik)
|
Cosmic gamma-ray line investigations |
Observing characteristic gamma ray lines from cosmic sources is both a
challenge and a great perspective: Operating such telescopes for gamma
rays in space means dealing with large instrumental backgrounds from
cosmic-ray interactions with the spacecraft and instrument. On the other
hand, gamma rays are penetrating messengers of supernova interiors, or
of diffuse nucleosynthesis throughout galaxies, or of annihilation of
positrons from various sources in the extended interstellar medium. With
56Ni and 44Ti radioactive decay gamma rays, we have presented
observational proof of such radioactivities being key energy sources of
supernovae. Beyond, the gamma-ray line characteristics help us to
constrain the spatial distribution and kinematics of the radioactive
products of nuclear fusion reactions deep in the supernova interior.
From distributed radioactive by-products of nucleosynthesis in the form
of the 26Al isotope, we could present a different view on the
core-collapse supernova rate in our Galaxy, which is based on data
originating throughout the extended Milky Way, rather than the solar
vicinity mostly. Finally, 511 keV line gamma rays have been measured
throughout the Milky Way's interstellar medium, as well as from a few
nearby galaxies. Galactic spectroscopy showed that a combination of
positron producers is probably responsible for seeding the ISM with
positrons, and propagation and slowing down is diffusing the trace of
the positrons. A microquasar flare revealed that such objects may be
copious contributors. Then, the annihilation line signal from dwarf
galaxy Ret II presented a surprise. With NAOJ collaborators we discussed
how this puzzle may fit into our nucleosynthesis source investigations
for heavy elements in a wider view. |
Cemsinan
Deliduman (Professor, Mimar Sinan Fine Arts University)
|
My current research interest is the gravity's
influence on the density of matter that shapes the nature of
astrophysical phenomena. Under this general title I am pursuing several
specific directions of research: I am interested with the significance
of extremely soft equations of state (EoS) for nuclear matter in
suprasaturation densities. In this respect I am aiming to determine in
a modified gravity theory, mass-radius relations of neutron stars with
very soft EoS agreeing with observations. Another line of research is
to understand astrophysical and cosmological phenomena, currently
attributed to existence of dark matter, solely in terms of interaction
of gravity with baryonic matter without assumption of dark matter. This
research proposes a new way of explaining the galactic flat rotation
curves, strong lensing by galaxies and galaxy clusters, weak lensing,
BBN mechanism and the nature of gravitational waves in certain
alternative theories of gravity. |
Yamac
Pehlivan (Professor, Mimar Sinan Fine
Arts University)
|
I am interested in several aspects of neutrinos
in Astronomy, Astrophysics, and Cosmology. This
includes their roles in core collapse supernovae, nucleosynthesis of
heavy elements, and the Big Bang Model. I am also interested in the
prospects of using neutrinos as an observational tool. Neutrinos are
the second most abundant particle species in the universe after
photons. But, unlike photons, they interact only weakly, which means
that their point of last scattering lies deep inside the object, not on
the surface as in the case of photons. Therefore neutrinos give us an
opportunity to look directly into the most extreme conditions that
exist in the Universe, such as a newly formed proto-neutron star at the
center of a core collapse supernova. Neutrino observatories such as the
Super Kamiokande are already looking to the Universe from this new
window, and I am working on how to discern neutrinos' message to us. |
Takuma
Suda (Doctor of Science, Research Associate, RESCEU)
|
The
research topic is the evolution of low- and
intermediate-mass (one to ~eight times the mass of the Sun) stars. The
chemical abundances of such stars are essential probes for
understanding the evolution of the universe as well as of our Galaxy.
The surface chemical composition of stars reflects the evolutionary
characteristics of individual stars. Therefore, the key component of
this topic is the comparisons of model stars with observations using
the surface chemical composition of stars. Stellar evolution theory can
be applied to various research topics such as mixing and
nucleosynthesis in stars and the Galactic chemical evolution in which I
am involved. |
Jun
Hidaka (Ph.D, Post Doctoral Research Associate, Meisei University)
|
Neutrino
Astrophysics |
My
current interest is on the
neutrino physics in gravitational core-collapse supernovae. I
especially focus on the study of fundamental roles of neutrino
oscillation in the explosion mechanism of supernovae. My study concerns
not only ordinary active neutrinos but also socalled sterile-
neutrinos, which takes presumably a key to novel energy transfer
mechanism for successful explosion. I also challenge to construct
rigorous formalism of quantum kinetic equation of neutrino transfer. |
Ko
Nakamura (Doctor of Science, Assistant Professor, Fukuoka University)
|
Core-Collapse
Supernovae and
Nucleosynthesis |
Stars
with mass more than
about eight solar masses cannot finally support themselves against
self-gravity, and culminate their evolution as core-collapse supernova
explosion. Shock wave produced from core bounce triggers explosion, and
subsequently heats and condensates stellar materials which serve to
provide hydrodynamic conditions for various nucleosynthetic processes
such as alpha-process (helium burning process), r-process (rapid
neutron-capture process), and neutrino-process (neutrino-induced
process). I study these processes in hydrodynamics numerical
simulations of the supernova explosions and nuclear network
calculations. |
Takami
Kuroda (Doctor of Science, Post Doctoral Research Associate, University
of Basel)
|
3D
Simulation of Core-Collapse
Supernovae and Gravitational Wave |
Massive
stars, heavier than
about eight solar mass, cannot support themselves against their
self-gravity at their final stage of stellar evolution and then core
collapse and subsequent explosion are triggered. The explosion is
called core-collapse supernova (CCSN). CCSNe affect strongly both
dynamical and chemical evolutions of galaxies, and therefore to reveale
their explosion mechanism is important. We study them in large-scale
numerical simulations in full three dimension by taking account of the
general relativistic effects and magnetic field effects. We also study
the gravitational wave forms and nucleosynthesis during CCSNe in order
to construct consistent CCSNe models both theoretically and
observationally. |
Dai
Yamazaki (Doctor of Science, Associate Professor, Ibaraki University)
|
Primordial
Magnetic Field, CMB, and
Cosmic Structure Formation |
Magnetic
fields of the
strength 0.1-1.0 micro Gauss have been observed in clusters of
galaxies. A primordial magnetic field (PMF) of order 1 nano Gauss,
whose field lines collapse as the cosmic structure forms, is one
possible explanation for such magnetic fields in galactic clusters. The
origin and detection of the PMF is, hence, a subject of considerable
interest in modern cosmology. We try to work out the effects of the PMF
on many physical processes in the Universe such as the cosmic microwave
background, the gravitational wave background, the large scale
structure formation, the Big-Bang nucleosynthesis, the cosmic
re-ionization, etc. in order to reconstruct cosmology and astrophysics
with the PMF. |
Shota
Shibagaki (Doctor of Science, Fukuoka University)
|
Supernova
and Nucleosynthesis |
Massive
stars culminate their
evolution by supernova explosions. They are the energetic phenomena in
the universe, where not only various hydrodynamic processes but even
the elementary particle processes such as the neutrino-nucleus
interactions take place. Almost all intermediate-to-heavy mass elements
up to actinides like uranium and thorium are produced in supernovae and
ejected into space. As such, supernovae are expected to be very
important and energetic astrophysical phenomena as the origin of heavy
elements.However, we still cannot reproduce their explosion nor explain
the solar-system abundance yet. My present research purpose is to solve
the explosion mechanism of core-collapse supernovae which leave a
proto-neutron star or a black hole from the view point of explosive
nucleosynthesis of heavy elements. |
Yutaka
Hirai (Doctor of Science, RIKEN)
|
Galactic
Chemical Evolution |
I
investigate the chemical
and dynamical evolution of galaxies in numerical simulations. ΛCDM
model implies hierarchical evolution of the Milky Way in terms of
merging small galaxies. According to this model, dwarf satellite
galaxies are expected to be the remnants from the very early stage of
forming Milky Galaxy. However, recent astronomical observations have
indicated that the metallicity distribution of some dwarf galaxies are
very different from that of the Milky Way. This is a piece of evidence
that the evolution of dwarf galaxies is quite unique, being totally
different from the Milky Way. I try to reveal the evolutionary process
of dwarf galaxies by taking account of the effects of star formation,
gas cooling, and feedback due to supernova explosions in numerical
simulations of chemical and dynamical evolution of dwarf satellite
galaxies of the Milky Way. |
Hirokazu
Sasaki (University of Tokyo, Doctor Course Student)
|
Neutrino Collective Oscillations |
In
core-collapse supernovae, after bounce, high
luminosity neutrinos are emitted from the proto-neutron star, and
around
100km from the center,non-linear effects are caused by forward
scatterings of self-interacting neutrinos. These effects are called
"Neutrino collective oscillations" because neutrinos oscillate
coherently in flavor space independent of their species and momentum.
Numerical simulations showed that by these effects,energy spectra of
neutrinos change dramatically and could affect the explosive
nucleosynthesis such as r-process,but the mechanism of these non-linear
effects are still unknown. So, I develop the calculation code, and try
to
study the mechanism of collective oscillations and apply to
nucleosynthesis. |
Kanji
Mori (University of Tokyo, Doctor Course Student)
|
Type Ia Supernova |
Type Ia
supernovae (SNe Ia) are thought to be a thermonuclear explosion of a
white dwarf (WD). It is famous that they are used as a standard candle
in cosmology. In addition to that, they produce a large amount of iron
group elements and play an important role in the galactic chemical
evolution. However, their progenitor is not well understood. In one
scenario called single-degenerate, it is attributed to accretion on a
WD whose mass is approaching the Chandrasekhar limit. In the other
scenario called double-degenerate, it is thought to be a WD-WD binary
merger. I aim to reveal the nature of the progenitor and SN Ia
explosion itself from the point of view of nucleosynthesis.
|
Yudong
Luo (University of Tokyo, Doctor Course Student)
|
Big Bang Nucleosynthesis with
Tsallis
Statistics |
Primordial
element synthesis process has been developed in computational code
while all the light element abundance prediction fits well with WMAP
observation except for Lithium-7. Recent research shows that Tsallis
statistics seems to be the suitable solution of it in homogeneous Big
Bang model. Since the physical meaning of this non-extensive
mathematical statistics is not clear so far, my current research is
trying to apply it into inhomogeneous Big Bang Model and explain the
physics behind this statistics. |
Basak
Ekinci (Mimar Sinan Fine Art University, Doctor Course Student)
|
NEUTRINO OSCILLATIONS IN THE CORE-COLLAPSE SUPERNOVAE |
Supernovae, the catastrophic explosions of stars, releases 99% of its gravitational binding
energy in terms of neutrinos. Although the neutrino interactions with matter are extremely
weak, they play an important role in a variety of astrophysical sites, such a dense medium
makes it possible for the neutrinos to interact with each other and matter. It causes the
flavor oscillations of neutrinos in astrophysical environments, such as the Sun or the
Supernova. These oscillations are different from neutrino oscillations in the vacuum. Our
study involves flavor oscillations of neutrinos and its effects in the core-collapse
supernovae.
|
Yuta Yamazaki (University of Tokyo, Master Course Student)
|
Chemical Evolution of r-Process Elements |
r-Process is a n-capture process which produces heavy elements up to Thorium or Uranium. Although r-process sites are increasingly becoming evident, r-process has not been fully clarified. r-Process sites are predicted to release gravitational waves of which observation will be developing in a next few decades, and next-generation giant telescopes (e.g., TMT) might reveal abundance patterns of distant and low-metallicity stars. By theoretical calculation of abundance patterns for all r-process isotopes, I aim to understand r-process sites and when they appear in cosmic history.
|